ESOT Calculations
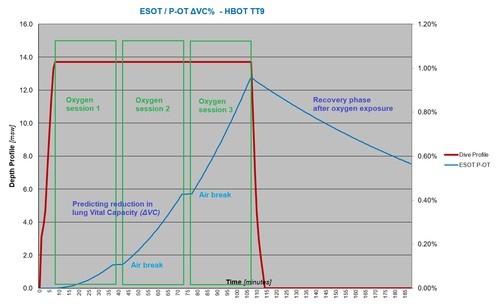
Defining Pulmonary Oxygen Toxicity risk in Subsea and Hyperbaric activities.
Text by Dennis Guichard
Humans are the weirdest things... our biological systems need oxygen to survive, but oxygen is actually a dose-dependent poison: too much of it can kill us. The study of human metabolic physiology and quite how oxygen affects us in the diving and hyperbaric environment is fascinating beyond belief.
One of the largest dilemmas with humans and anything to do with our biology is how we are each quite bioindividual. No two of us are quite alike. What ‘works’ for or impacts one isn’t necessarily going to ‘work’ for or impact the next. So, the best we can fairly do is consider mean-impact on the masses but remain mindful that many of us may be outliers to that mean.
In terms of decompression theory or oxygen toxicity risk, that means that some of us might bend, or experience an oxygen toxicity event, whilst being well within conventional norms.
Text by Dennis Guichard
Humans are the weirdest things... our biological systems need oxygen to survive, but oxygen is actually a dose-dependent poison: too much of it can kill us. The study of human metabolic physiology and quite how oxygen affects us in the diving and hyperbaric environment is fascinating beyond belief.
One of the largest dilemmas with humans and anything to do with our biology is how we are each quite bioindividual. No two of us are quite alike. What ‘works’ for or impacts one isn’t necessarily going to ‘work’ for or impact the next. So, the best we can fairly do is consider mean-impact on the masses but remain mindful that many of us may be outliers to that mean.
In terms of decompression theory or oxygen toxicity risk, that means that some of us might bend, or experience an oxygen toxicity event, whilst being well within conventional norms.
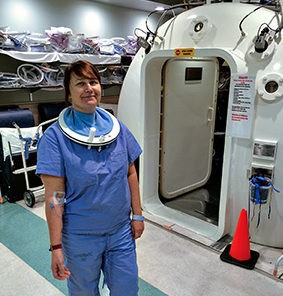
Whilst conversely, some of us may be able to push the boundaries to some level of extreme without impact. Although our biology isn’t just individualistic; it’s also varied such that what we might get away with today is not the same as what might hit us tomorrow. Humans can be complicated beyond belief.
The magic of math and science, however, is that we can actually calculate and predict the mean risk and probability of both Central Nervous System (CNS-OT) and Pulmonary Oxygen Toxicity (P-OT) to some degree. Although oxygen toxicity prediction is what we’d fairly call ‘stochastic’, in that it has a ‘random probability distribution that may be analysed statistically but may not be predicted precisely’.
The elevated oxygen load that our bodies might have to cope with affects different biological organs at different levels of exposure. With anything between about 0.5-1.2 bar ppO2 exposure, our lungs seem to be one of the primary ‘organs of decay’. Between these relatively low-level limits, the Reactive Oxygen Species (ROS), generated as an excessive by-product of oxygen metabolism, predominantly damage the alveolar-capillary barrier in our lungs over time, leading to inflammation.
Plasma leaks into our alveoli, and our critical gas exchange interface is hindered until we literally drown in our own plasma. And it’s that time-dose dependant reduction (and limits) in pulmonary Vital Capacity (ΔVC) that we can calculate and predict.
The pulmonary effects of diving aren’t of course just restricted to elevated ppO2 levels when other contributing factors can also cause damage to lung membranes. Such cellular damage and inflammation also lead to secondary alveolar fibrosis and further loss of diffusion capacity.
The production of excess ROS ceases as soon as we stop breathing the elevated ppO2 mixture or take an air break between dose exposures.
The cellular alveoli damage will immediately start to recover, although the inflammation process can still continue for many hours (as much as a 5-hour delay predicted by Barbara Shykoff).
Various mathematical models have been proposed over the decades to try and help predict these detrimental effects, all of which have their benefits and limitations. There’s no perfect science.
As long as I can remember, our dive books have always taught us to refer to the Unit Pulmonary Toxic Dose (UPTD) methodology, also commonly referred to as the Oxygen Toxicity Unit (OTU), for calculating Pulmonary Oxygen Toxicity (P-OT) limits. The reality, however, is that those limits, developed in 1970, were never actually based on tested and validated wet dive exposures. They also didn’t make any provision for partial or complete recovery between exposures. They were an invaluable start to understanding the oxygen toxicity malady but far from perfect.
One of the real-world challenges I’ve long battled with is trying to predict oxygen toxicity risk and probability in a hyperbaric environment where we have patients and/or injured divers breathing 100% oxygen at as much as 2.4-2.8 bar ppO2 for extended periods of time.
How much oxygen is too much oxygen, and how do we understand and ensure we’re not risking more harm (i.e., oxygen toxicity symptoms) than good with how we manage treatments? It should be something we can easily calculate, predict, and manage with some level of accuracy.
In the 1980s, a REPEX methodology (building on the UPTD/OTU system) was published that supposedly facilitated recovery and multi-day exposures, but it was never validated in divers. Harabin et al published a paper in 1985 with their approach to assessing P-OT, as did Vann in 1988 with another.
In 2002 (updated in 2019 & 2020), Arieli et al published a fresh new methodology for calculating both P-OT and CNS-OT, called the ‘Power Equation’. So-called because of the identified time-squared non-linear regressive relationship with various forms of measurable oxygen toxicity symptoms and the known rate of hydroxyl radical production (a precursor to ROS). The Arieli methodology also includes a formula for calculating recovery between and after exposures.
P-OT is typically measured in terms of the reduction in lung vital capacity (ΔVC), brought about by damage and inflammation to the alveolar membranes. The US Navy oxygen exposure limits require no more than a 2% reduction in Vital Capacity and no more than a 10% decrement as a maximum limit. Using these limits, the Arieli methodology helps define oxygen exposure and time frameworks that we can adhere to in minimising risk.
In 2015, the Shykoff ‘incidence-time model’ methodology (that I actually really like) was published, which also included a recovery model. It, however, limited consideration to a narrow exposure range of between just 1.3-1.4 bar ppO2 (so ideal for rebreather diver application) and is based on a different recovery approach to Arieli, giving different predictions.
One of the struggles I’ve found, however, in trying to assess P-OT and CNS-OT risk when treating patients is when we have fluctuating profiles with variable ppO2, time, & air break recovery exposures. The Arieli Power Equation is simple enough for end-point calculation with fixed ppO2 and singular overall time exposures but is immensely difficult to calculate for more complex multi-factorial exposures.
Published only recently in June 2023, the ‘Equivalent Surface Oxygen Time’ (ESOT) methodology enables us to predict changes in ΔVC and recovery with ease. Based directly on a ‘simplification’ of the Arieli Power Equation, it is a fresh new approach with which I’m able to plot out literally a second-by-second analysis of predictive ΔVC impact on complex patient treatments (see accompanying graph on previous page).
ESOT has tangible practical meaning in that it applies Lambertsen’s UPTD concept of expressing lung damage as ‘minutes on surface oxygen’ - 1 ESOT is thus ‘simply’ also the hyperoxic exposure reached after breathing 100% O2 for 1 minute at 1 atmosphere.
I’ve calculated exposures using both the Power Equation and the ESOT methodologies, and they do align perfectly. The ESOT, however, is quite simple to plot, even for a layman like me using an elementary Excel spreadsheet. The ESOT methodology is based on a previous foundation of real dive testing in both dry and wet exposure and a clearer modern-day understanding of ROS biological activity.
The ESOT approach to plotting P-OT has been adopted and formally published by the world’s leading hyperbaric and pulmonary experts on the ‘Diving Medical Advisory Committee’ (which serves the commercial and saturation diving industry worldwide), as their official ‘DMAC 35 Guidance Note’ - for more, refer to: www.dmac-diving.org.
The magic of math and science, however, is that we can actually calculate and predict the mean risk and probability of both Central Nervous System (CNS-OT) and Pulmonary Oxygen Toxicity (P-OT) to some degree. Although oxygen toxicity prediction is what we’d fairly call ‘stochastic’, in that it has a ‘random probability distribution that may be analysed statistically but may not be predicted precisely’.
The elevated oxygen load that our bodies might have to cope with affects different biological organs at different levels of exposure. With anything between about 0.5-1.2 bar ppO2 exposure, our lungs seem to be one of the primary ‘organs of decay’. Between these relatively low-level limits, the Reactive Oxygen Species (ROS), generated as an excessive by-product of oxygen metabolism, predominantly damage the alveolar-capillary barrier in our lungs over time, leading to inflammation.
Plasma leaks into our alveoli, and our critical gas exchange interface is hindered until we literally drown in our own plasma. And it’s that time-dose dependant reduction (and limits) in pulmonary Vital Capacity (ΔVC) that we can calculate and predict.
The pulmonary effects of diving aren’t of course just restricted to elevated ppO2 levels when other contributing factors can also cause damage to lung membranes. Such cellular damage and inflammation also lead to secondary alveolar fibrosis and further loss of diffusion capacity.
The production of excess ROS ceases as soon as we stop breathing the elevated ppO2 mixture or take an air break between dose exposures.
The cellular alveoli damage will immediately start to recover, although the inflammation process can still continue for many hours (as much as a 5-hour delay predicted by Barbara Shykoff).
Various mathematical models have been proposed over the decades to try and help predict these detrimental effects, all of which have their benefits and limitations. There’s no perfect science.
As long as I can remember, our dive books have always taught us to refer to the Unit Pulmonary Toxic Dose (UPTD) methodology, also commonly referred to as the Oxygen Toxicity Unit (OTU), for calculating Pulmonary Oxygen Toxicity (P-OT) limits. The reality, however, is that those limits, developed in 1970, were never actually based on tested and validated wet dive exposures. They also didn’t make any provision for partial or complete recovery between exposures. They were an invaluable start to understanding the oxygen toxicity malady but far from perfect.
One of the real-world challenges I’ve long battled with is trying to predict oxygen toxicity risk and probability in a hyperbaric environment where we have patients and/or injured divers breathing 100% oxygen at as much as 2.4-2.8 bar ppO2 for extended periods of time.
How much oxygen is too much oxygen, and how do we understand and ensure we’re not risking more harm (i.e., oxygen toxicity symptoms) than good with how we manage treatments? It should be something we can easily calculate, predict, and manage with some level of accuracy.
In the 1980s, a REPEX methodology (building on the UPTD/OTU system) was published that supposedly facilitated recovery and multi-day exposures, but it was never validated in divers. Harabin et al published a paper in 1985 with their approach to assessing P-OT, as did Vann in 1988 with another.
In 2002 (updated in 2019 & 2020), Arieli et al published a fresh new methodology for calculating both P-OT and CNS-OT, called the ‘Power Equation’. So-called because of the identified time-squared non-linear regressive relationship with various forms of measurable oxygen toxicity symptoms and the known rate of hydroxyl radical production (a precursor to ROS). The Arieli methodology also includes a formula for calculating recovery between and after exposures.
P-OT is typically measured in terms of the reduction in lung vital capacity (ΔVC), brought about by damage and inflammation to the alveolar membranes. The US Navy oxygen exposure limits require no more than a 2% reduction in Vital Capacity and no more than a 10% decrement as a maximum limit. Using these limits, the Arieli methodology helps define oxygen exposure and time frameworks that we can adhere to in minimising risk.
In 2015, the Shykoff ‘incidence-time model’ methodology (that I actually really like) was published, which also included a recovery model. It, however, limited consideration to a narrow exposure range of between just 1.3-1.4 bar ppO2 (so ideal for rebreather diver application) and is based on a different recovery approach to Arieli, giving different predictions.
One of the struggles I’ve found, however, in trying to assess P-OT and CNS-OT risk when treating patients is when we have fluctuating profiles with variable ppO2, time, & air break recovery exposures. The Arieli Power Equation is simple enough for end-point calculation with fixed ppO2 and singular overall time exposures but is immensely difficult to calculate for more complex multi-factorial exposures.
Published only recently in June 2023, the ‘Equivalent Surface Oxygen Time’ (ESOT) methodology enables us to predict changes in ΔVC and recovery with ease. Based directly on a ‘simplification’ of the Arieli Power Equation, it is a fresh new approach with which I’m able to plot out literally a second-by-second analysis of predictive ΔVC impact on complex patient treatments (see accompanying graph on previous page).
ESOT has tangible practical meaning in that it applies Lambertsen’s UPTD concept of expressing lung damage as ‘minutes on surface oxygen’ - 1 ESOT is thus ‘simply’ also the hyperoxic exposure reached after breathing 100% O2 for 1 minute at 1 atmosphere.
I’ve calculated exposures using both the Power Equation and the ESOT methodologies, and they do align perfectly. The ESOT, however, is quite simple to plot, even for a layman like me using an elementary Excel spreadsheet. The ESOT methodology is based on a previous foundation of real dive testing in both dry and wet exposure and a clearer modern-day understanding of ROS biological activity.
The ESOT approach to plotting P-OT has been adopted and formally published by the world’s leading hyperbaric and pulmonary experts on the ‘Diving Medical Advisory Committee’ (which serves the commercial and saturation diving industry worldwide), as their official ‘DMAC 35 Guidance Note’ - for more, refer to: www.dmac-diving.org.
Posted in Alert Diver Southern Africa, Research
Posted in Oxygen Administration, Oxygen Toxicity, HBO
Posted in Oxygen Administration, Oxygen Toxicity, HBO
Categories
2024
February
March
April
May
October
My name is Rosanne… DAN was there for me?My name is Pam… DAN was there for me?My name is Nadia… DAN was there for me?My name is Morgan… DAN was there for me?My name is Mark… DAN was there for me?My name is Julika… DAN was there for me?My name is James Lewis… DAN was there for me?My name is Jack… DAN was there for me?My name is Mrs. Du Toit… DAN was there for me?My name is Sean… DAN was there for me?My name is Clayton… DAN was there for me?My name is Claire… DAN was there for me?My name is Lauren… DAN was there for me?My name is Amos… DAN was there for me?My name is Kelly… DAN was there for me?Get to Know DAN Instructor: Mauro JijeGet to know DAN Instructor: Sinda da GraçaGet to know DAN Instructor: JP BarnardGet to know DAN instructor: Gregory DriesselGet to know DAN instructor Trainer: Christo van JaarsveldGet to Know DAN Instructor: Beto Vambiane
November
Get to know DAN Instructor: Dylan BowlesGet to know DAN instructor: Ryan CapazorioGet to know DAN Instructor: Tyrone LubbeGet to know DAN Instructor: Caitlyn MonahanScience Saves SharksSafety AngelsDiving Anilao with Adam SokolskiUnderstanding Dive Equipment RegulationsDiving With A PFOUnderwater NavigationFinding My PassionDiving Deep with DSLRDebunking Freediving MythsCryptic FiishImmersion Pulmonary OedemaSwimmer's EarMEMBER PROFILE: RAY DALIOAdventure Auntie: Yvette OosthuizenClean Our OceansWhat to Look for in a Dive Boat
2023
January
March
Terrific Freedive ModeKaboom!....The Big Oxygen Safety IssueScuba Nudi ClothingThe Benefits of Being BaldDive into Freedive InstructionCape Marine Research and Diver DevelopmentThe Inhaca Ocean Alliance.“LIGHTS, Film, Action!”Demo DiversSpecial Forces DiverWhat Dive Computers Don\'t Know | PART 2Toughing It Out Is Dangerous
April
July
August